Atoms, Molecules, and More with the Molecular Workbench
Nobel Prize winner Richard Feynman once said, “If, in some cataclysm, all of scientific knowledge were to be destroyed, and only one sentence passed on to the next generations of creatures, what statement would contain the most information in the fewest words? I believe it is the atomic hypothesis . . . that all things are made of atoms—little particles that move around in perpetual motion, attracting each other when they are a little distance apart, but repelling upon being squeezed into one another. In that one sentence, you will see, there is an enormous amount of information about the world, if just a little imagination and thinking are applied.”1
The wisdom of this great scientist highlights the fundamental importance of atoms as the building blocks of the world and the knowledge about them as a foundation of science. Science educators agree with him. The science of atoms and molecules constitutes a substantial part of the science content standards2 and even more so in the new frameworks,3 from the structure of atoms to the molecular basis of heredity. This body of knowledge and skills, known as molecular literacy and molecular reasoning, is increasingly important as science has advanced to the point that the research and development of atomic-scale systems and technologies holds the key to solving many critical problems today.
But what happens at the atomic level is difficult for students to imagine and molecular literacy focuses on unfamiliar concepts. Due to the lack of teaching tools in the past, these concepts were often taught as factual knowledge that students had to accept and memorize. Fortunately, computer technology has provided a revolutionary way to teach them.
The Molecular Workbench (MW) software is one of the most advanced tools for teaching and learning the science of atoms and molecules. MW includes a set of computational engines that accurately simulate atomic motions, quantum waves, and atomic-scale interactions based on fundamental equations and laws in physics. These engines render highly expressive dynamic visualizations of atomic-scale phenomena on the computer screen and provide rich user interfaces for exploration. MW empowers students to learn through conducting graphical “computational experiments”4 to investigate ideas otherwise untestable in classrooms. This capacity provides opportunities for inquiry and effective pathways to molecular literacy and reasoning skills.
Physics | Chemistry | Biology | Nanotechnology | Biotechnology |
---|---|---|---|---|
Heat & Temperature | Atomic Structure | Molecular Recognition | Nanostructures | X-Ray Crystallography |
Spectroscopy | Kinetic Molecular Theory | Lipids | Nanomachines | DNA Hybridization |
Electrostatics | Gas Laws | Carbohydrates | Self Assembly | Southern Blot |
Electricity | Phase Change | Proteins | Scanning Tunneling Microscopy | ELISA |
Semiconductors | Intermolecular Interactions | Nucleic Acids | Atomic Layer Chemical Vapor Deposition | Fluorescent Tagging |
Quantum Tunneling | Molecular Geometry | Genetic Code | Sputtering | FACS |
Quantum Diffraction | Solubility | Transcription | Electrophoresis | |
Excited States & Photons | Polymerization | Translation | Mass spectrometry | |
Photoelectric Effect | Chemical Reactions | Photosynthesis |
Changing how science is taught
If a picture is worth a thousand words, a visual simulation is good for at least 10,000! Molecular Workbench significantly lowers the barrier for learning and teaching abstract concepts. Teachers can demonstrate a concept with a salient dynamic visualization without intimidating students with obscure terminology or difficult mathematics.
For example, one study conducted by the University of Illinois at Chicago showed that the seemingly complicated idea of molecular self-assembly can be taught to elementary school students if dynamic visualizations from MW are used to illustrate the key points — that molecules are moving all the time, they are “sticky” in some way, and their shapes must match for assembly. Another example is quantum tunneling. The traditional approach to teaching this concept is through mathematical analysis that few students can master. MW provides a new way to investigate how different properties affect tunneling without using any equations, making the concept accessible to more students.
Research studies of diverse students ranging from middle grades through college demonstrate that students who use well-designed MW activities gain understanding of atomic-scale phenomena and can transfer this knowledge to new contexts effectively.
A rich collection of curriculum materials
One of the unique strengths of MW is its ability for curriculum developers and instructional designers to create curriculum activities that lead students through well-planned investigations and explorations.
A sequence of pages — containing text, multimedia, models, simulations, games, graphs, assessments, and networking capabilities — progressively develops a concept. Students can answer questions, save their work in a Web portfolio, share models with collaborators, create electronic reports, and submit them for grading. We have developed hundreds of activities for biology, chemistry, physics, biotechnology, and nanotechnology. They’re all freely available online.
The deeply digital textbook of tomorrow
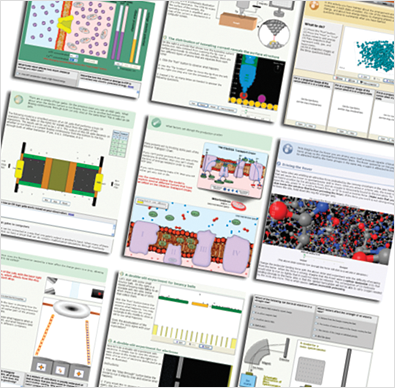
These model-based activities represent what could become chapters in a nextgeneration digital textbook. This deeply digital vision of textbooks differs from most of the e-texts available today by including interactive explorations of models that replace static illustrations. This approach can change what students learn, making it possible to teach deeper concepts that have greater explanatory power. Thus, the textbook of tomorrow will be much more than textbooks transferred to computers — they will permit students to learn more, more deeply.
Molecular Workbench Facts
Molecular Workbench was made possible by a succession of National Science Foundation grants. It has been downloaded over 800,000 times by users worldwide. In June it was awarded a Science Prize for Online Resources in Education (SPORE). The SPORE prize was established by the American Association for the Advancement of Science to “encourage innovation and excellence in education, as well as to encourage the use of high-quality on-line resources by students, teachers, and the public.”
1. Feynman, R. P., Leighton, R. D., & Sands, M. (1963). The Feynman lectures on physics. Menlo Park: Addison-Wesley.
2. National Science Education Standards, www.nap.edu/openbook.php?record_id=4962
3. See www7.nationalacademies.org/bose/Standards_Framework_Homepage.html
4. Computational Experiments for Science Education, www.sciencemag.org/content/332/6037/1516.ful
Charles Xie (qxie@concord.org) is the developer of the Molecular Workbench software.
Robert Tinker (rtinker@concord.org) is the founder of the Concord Consortium.