Teaching Future Nanoscientists in Today’s Classrooms
The new field of nanoscience promises to solve many critical problems in medicine, electronics, and energy production. Advancements in nanoscience and nanotechnology will require creativity from scientists and technologists for many generations, which puts nanoscience education at a pivotal point in the field of science education.
Nanoscience is the science of controlling and manufacturing matter at the atomic and molecular scale. There has been considerable investment in nanoscience education in K-12 classrooms since the inception of the National Nanotechnology Initiative in 2001. However, it is still unclear how nanoscience should be taught at the secondary level. One unanswered question is: Should nanoscience be treated as a new discipline or should it be spread across existing disciplines?
Purists vs. pragmatists
The purists propose an independent course. And there are good reasons for such a recommendation. Nanoscience can be considered a foundational science from which large portions of physical and biological sciences are derived. The reason that we put different parts of nanoscience into different branches of science is mostly artificial. All natural and synthesized nanoscale systems are made of electrons, atoms, and molecules that obey the same set of rules. They differ only in structures and functions that can be explained by applying those rules. Occam’s razor, which assumes the simplest solution is usually correct, seems to suggest that students might be better off if nanoscience were a prerequisite for physical and biological sciences.
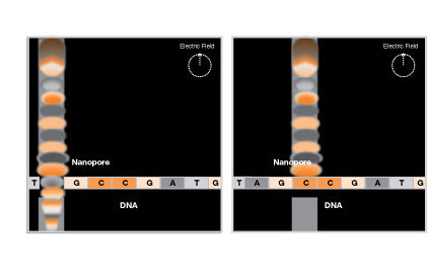
The pragmatists plead for a conservative treatment that views nanoscience as an applied science rather than as a basic science. Arguably, many breakthroughs in nanoscience can be proclaimed triumphs of applying physics, chemistry, biology, or some combination of the three to problems at the nanoscale. For this reason, it appears natural to break up nanoscience into a collection of supplementary modules that can be readily plugged into the current curricular frameworks. In this way, students are exposed to cutting-edge nanoscience concepts without compromising the integrity of the current science curricula and the continuity of the teaching tradition.
Both sides are paradoxical. Advocating for an independent course suffers from the reality that young students typically lack the abstract thinking skills needed to reason from fundamental principles. Ironically, these skills are usually acquired only after learning the more factual physical and biological sciences. Without an understanding of scientific concepts at the macroscopic level that connect to their everyday lives, students may not be able to make the theoretical leap to the nano scale.
The argument for integrating nanoscience into the current science curricula has a fundamental flaw as it raises the very barriers between disciplines that scientists and engineers working in the nano field strive to eliminate. By describing nanoscience as an extension or an application of a discipline, educators risk failing to deliver an important message from the frontier researchers—that the nature of nanoscience is multidisciplinary, the artificial barriers between sciences need to be broken down, and students should have this updated mindset as early as possible.
Since there is no perfect solution, these two divergent approaches to nanoscience education will coexist for a long time. On the one hand, there are college-level courses dedicated to teaching nanoscience systematically, which will gradually influence secondary level practice. On the other hand, as the current science curricula incorporate more and more nanoscience content, we may reach a point where the mission of nanoscience education has been quietly accomplished under the guise of other disciplines.
In any case, it is time to roll up our sleeves and get to work on creating ways to make nanoscience education more effective, regardless of which path it takes.
Teaching quantum mechanics
One of the hardest challenges in nanoscience education is the teaching of quantum mechanics. Since quantum mechanics rules the nanoscale world, its importance cannot be overestimated. But the quantum world is totally counterintuitive and sometimes even downright spooky. Yet, it is undeniably real and ubiquitous. IBM Fellow Donald M. Eigler, Ph.D., has said of his work with colleagues who discovered a way to transport information based on the wave nature of electrons, “We have become quantum mechanics—engineering and exploring the properties of quantum states. We’re paving the way for the future nanotechnicians.” Indeed, many technological advances would not have been possible without a solid understanding of quantum mechanics.
The Electron Technologies project, funded by the National Science Foundation, has developed a quantum dynamics engine for simulating the quantum behavior of the nanoscale world. Based on numerically solving the time-dependent Schrödinger equation, this engine is capable of simulating a wide variety of dynamic quantum processes: bound state, excited state, quantum transition, the formation of a covalent bond, chemical polarity, field-induced polarization, ionization, diffraction, interference, tunneling, quantum transport, and more. It is fascinating to see that these seemingly disparate concepts in physics and chemistry emerge from quantum dynamics simulations that are based on a few basic assumptions— that electrons are represented by moving waves and that waves interact with each other and nuclei through electrostatic interactions. In fact, the quantum explanation of chemistry is among the greatest scientific discoveries in the 20th century, as evidenced by several Nobel Prizes awarded to computational chemists.
By making counterintuitive and mysterious phenomena more understandable and approachable, we are paving the way for future nanoscientists and nanotechnicians.
The quantum dynamics engine is part of the NSF-funded Molecular Workbench software and has been used to produce simulations for several interactive modules for the Electron Technologies project. These modules cover nanoscience lessons that teach concepts such as scanning tunneling microscopy and nanoelectronics. For example, Figure 1 shows how quantum dynamics simulations can be used to explain the principle of rapid DNA sequencing using a solid-state nanopore. Developed by the Harvard Nanopore Group, this innovative technology works like a scanning tunneling microscope. A denatured DNA strand steps through an electrically biased nanopore electrophoretically. The passing base alters the tunneling current across the nanopore, generating a signal that tells its type. Thus, the DNA code can be cracked while the strand translocates through the pore.
Of course, quantum dynamics simulations and molecular dynamics simulations provided by the Molecular Workbench software cannot solve the paradox of nanoscience education. But they do provide visible and tangible solutions that lower learning barriers. By making counterintuitive and mysterious phenomena more understandable and approachable, the Concord Consortium is paving the way for future nanoscientists and nanotechnicians. Purists and pragmatists alike will celebrate as nanoscience education makes leaps and bounds—at the nano scale, of course.