Integrating Technology and Science: Preparing Students for the World They Live In
As science educators our goal is to help students engage in and develop practices for understanding the natural world. But what about the built world, the human-made environment—the one that students spend much of their day-to-day lives in? We teach science for many reasons: to prepare students to develop thinking skills and understand the world around them, to engage as citizens in their communities, and to enter the workforce. We should teach about technology for the same reasons.
Our modern technological world is rapidly changing, and most people know little about the technological forces that are reshaping their work and home life. From a household smart thermostat to autonomous vehicles, from an Amazon Dash button to the “Internet of Behaviors,” our world is sensed, interpreted, and algorithmically controlled by technologies we didn’t create ourselves. Many technologies on the horizon (e.g., “deepfakes” and art generated by machine learning algorithms, gene editing with CRISPR, artificial general intelligence) will fundamentally change how we live and relate to one another. Yet school does little to prepare students to understand—let alone change—this increasingly technological world.
Moreover, nearly every future job is going to require unprecedented competence in working with technology. Workers will need a complex mix of creativity, knowledge, and specific competencies, such as interpreting data from sensor networks or training machine learning algorithms. Whether or not a student ultimately pursues a career in STEM, it is nonetheless empowering to be able to understand, adapt, and change the built world. And because science and technology are deeply entangled, science classrooms are an ideal place to look for opportunities for teaching with and about technology.
How do science and technology progress together?
Science education includes learning about how scientific knowledge is produced. Part of understanding “the nature of science” entails understanding the relationship between scientific and technological progress. One common belief is that science creates knowledge and technology applies it. But this is an oversimplification. Scholars who study scientific progress, both historically and philosophically, describe a much more complicated and nuanced story. Science itself is deeply technological.
If science transforms the built world to produce knowledge, technology begins with knowledge and produces material artifacts; it “realizes” ideas in the world. To create useful, reliable scientific insights, these two processes must happen simultaneously, in a back-and-forth dance. Andrew Pickering’s The Mangle of Practice details how technical and design work in the laboratory are as necessary to scientific progress as developing scientific models.* As scientists improve their tools and techniques for creating phenomena or taking measurements, their understanding of technologies co-evolves with their understanding of the natural world.
In the mid-1800s, physicists Hippolyte Fizeau and Léon Foucault made a series of non-astronomical measurements of the speed of light. Their initial experimental design involved a light source and a faraway mirror, with a spinning toothed gear between them. One of the most difficult problems with the measurement—and a primary source of uncertainty in the results—was entirely technical: they needed a motor that could spin with a precisely known rotational speed. Foucault enlisted the help of Louis François Clément Breguet—a watchmaker turned physicist—to develop an improved fixed-speed motor. Later iterations of the motor apparatus allowed them to make increasingly accurate and precise measurements.
Indeed, many important contributions to science are technical rather than purely theoretical. For example, the Davenport Motor (the first DC electric motor built in the U.S.) was made by the blacksmith Thomas Davenport who, with little formal education, made use of a connection between electricity and magnetism that hadn’t been theorized. Likewise, the helical model of DNA could not have been developed without Rosalind Franklin’s work on X-ray crystallographic imaging techniques. And consider the discovery of the Higgs Boson at the Large Hadron Collider (LHC). This massive technological undertaking involved thousands of scientists and engineers. A fraction of the team developed particle theory; many more built detectors, developed computational algorithms, or figured out how to cool the LHC’s superconducting magnets. It’s clear we need to broaden our view of science practice to include work with and development of technology.
Teaching science and technology together, at last
As standards have pushed to have science education reflect the authentic processes of science, they have incorporated “practices” as a way of learning about the world and developing scientific knowledge. But many science classroom practices emphasize talk—through discussions, argumentation, and literacy, including reading and writing about science. It is equally important to consider how students’ engagement in science practice can develop their technical knowledge and technical skills.
So how should science education change as the modern world changes? We need a model of science education that can effectively respond to technological change as well as new demands for teaching about technology. We should look for opportunities to integrate science and technology education in ways that are complementary, where the use of technology is integrated into science practice in authentic ways, and where technical knowledge is valued as a product of scientific activity.
Integrating science and technology learning
Scientists often use specialized hardware and software to work with systems for data acquisition and control. The hardware consists of electronics that connect to and sample voltages or currents from connected sensors and turn those into numerical readings. The software (like the popular LabVIEW) controls the sampling, processing, and storage of these data, as well as the actuation of connected devices—for instance, to drive a piezoelectric crystal or a current through a heating or cooling device. These systems can be used to collect experimental data, create experimental conditions, or control an experiment.
Our InSPECT research project, funded by the National Science Foundation, focuses on integrating science and technology learning by creating tools for data acquisition and control. The project engages students in using these technologies to do more authentic experiments involving data collection and analysis. InSPECT makes use of custom-made sensor hardware for creating data, and a LabVIEW-like programming environment for data processing, visualization, and storage, and for the logical control of actuators. Students use these scientific tools to make measurements and analyze data, and learn about computing and sensor hardware at the same time.
InSPECT developed a three-week curriculum investigating the biological processes in plants and animals that produce or remove carbon dioxide from the atmosphere. Throughout the curriculum, students use our custom Dataflow data acquisition and control hardware and software to do several hands-on laboratory experiments to investigate photosynthesis and cellular respiration (Figure 1). They use sensors to measure CO2 levels in the atmosphere and in controlled environments, while programming LED lamps to turn off and on to stimulate photosynthesis (Figure 2).
The instructional sequence was designed such that students learn about science and technology in parallel. We wanted students to engage in authentic science practices to develop their understanding of biology, learn about modern sensor-based technologies including Internet of Things (IoT) sensor devices, and gain technical skills in programming for data collection and control. Four underlying design principles guided our work.
Using dual learning progressions. Each activity has two sets of learning goals, one about science practices and content and another about the technology. For instance, students are first introduced to the topic of carbon dioxide in the atmosphere when they inspect graphs of CO2 levels from their classroom. They observe features of the graphs—when the Internet shut down, when the custodian was in the room, when students were in class. This helps them start to think not only about carbon dioxide and where it comes from and where it goes, but also about all the other things that sensors can “make visible” about the world around us.
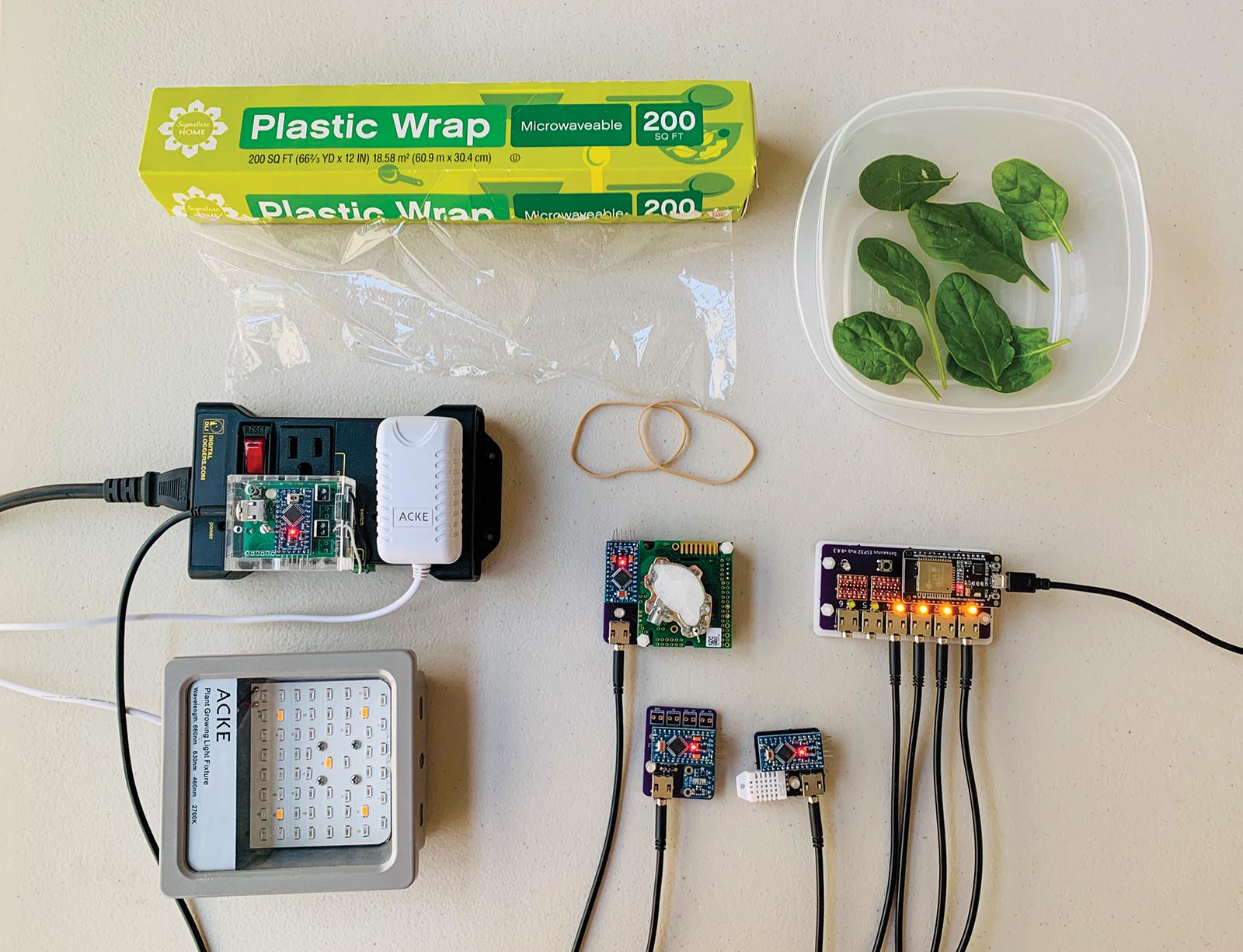
Introducing sensors as technology. After seeing graphs of the classroom CO2 levels, students make their own measurements. They notice that their sensors all have different CO2 readings of the classroom, which creates an opportunity to discuss sources of variation in measurement and issues of accuracy and precision. When learning to control actuators, they use the technology to create automatic alarms that turn on when the CO2 in the classroom reaches unhealthy levels. When experimenting with the hardware during free time, many students built their own “smart devices,” such as an automatic nightlight or a “clapper.”
Redesigning hands-on labs to problematize measurement. In contrast to most sensor-based labs, InSPECT labs give students opportunities to learn about the technology they’re using to produce their data. For example, the sensors—and their limitations—become central to a redesigned respiration lab, in which students devise ways to measure the concentration of carbon dioxide in their breath both before and after exercise. However, the concentration of CO2 in undiluted breath is much higher than the sensors can measure directly. Students learn more about what the sensors actually measure and how, then design their measurements around these limitations. For instance, some popped small balloons of exhaled breath into a larger bag of air, while others blew through a straw above the surface of the sensor.
This variation in methods is an indication that students are engaged in scientific thinking about experimental design themselves. And when they then use the sensors to create their own data, they begin to wonder more about them: How do the sensors work inside? How fast can they make measurements? What else might affect the values I see?
Modifying hands-on labs to include programming and control. After a series of activities in which students come to understand photosynthesis and respiration in plants and animals as processes that produce or consume carbon dioxide, they create a final project that puts both their scientific and technical knowledge to use. Students design a way to stabilize the carbon dioxide levels in a closed “ecosphere” container. Some students programmed a light to turn on when the CO2 levels in the ecosphere are too high; the plant matter inside then photosynthesizes and the CO2 levels dropped. In this way, students are introduced to scientific practices of using technology to control experimental variables. Depending on how they set up their system and their program, they saw different behaviors of their CO2 control system.
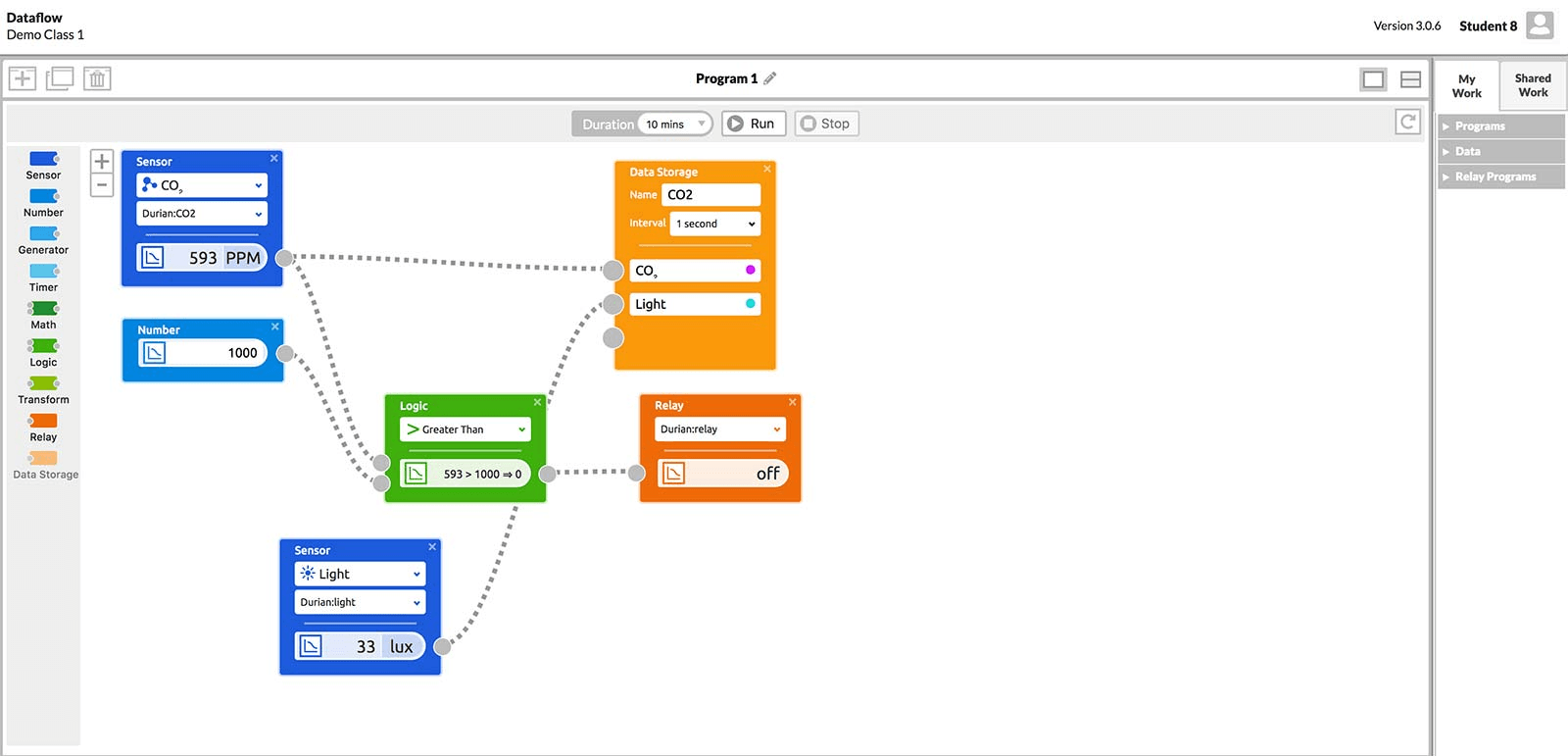
Conclusion
During classroom observations, we have witnessed students get inspired by the technology. This shouldn’t surprise us—just like the natural world, technology in the built world can be fascinating. While technology education has its own purpose and goals, learning with and about technology can also serve as an onramp to science practice.
For science and technology integration to work, students must be able to learn about the technologies they use to create scientific knowledge. To ensure that these technologies are relevant to modern work and life, we should leverage connections between scientific tools and the “everyday” technologies in our modern world. The InSPECT project builds on the synergy between the tools used for data acquisition and control and the technologies underlying modern IoT devices (systems of sensors, computers, and actuators). We are excited by the potential of technology integration in the science classroom to foster creativity and competencies, along with both scientific and technical knowledge.
* Pickering, A. (2010). The mangle of practice: Time, agency, and science. Chicago: University of Chicago Press.
Lisa Hardy (lhardy@concord.org) is a research associate.
This material is based upon work supported by the National Science Foundation under grant DRL-1640054. Any opinions, findings, and conclusions or recommendations expressed in this material are those of the author(s) and do not necessarily reflect the views of the National Science Foundation.