Modeling Plate Tectonics for Learning
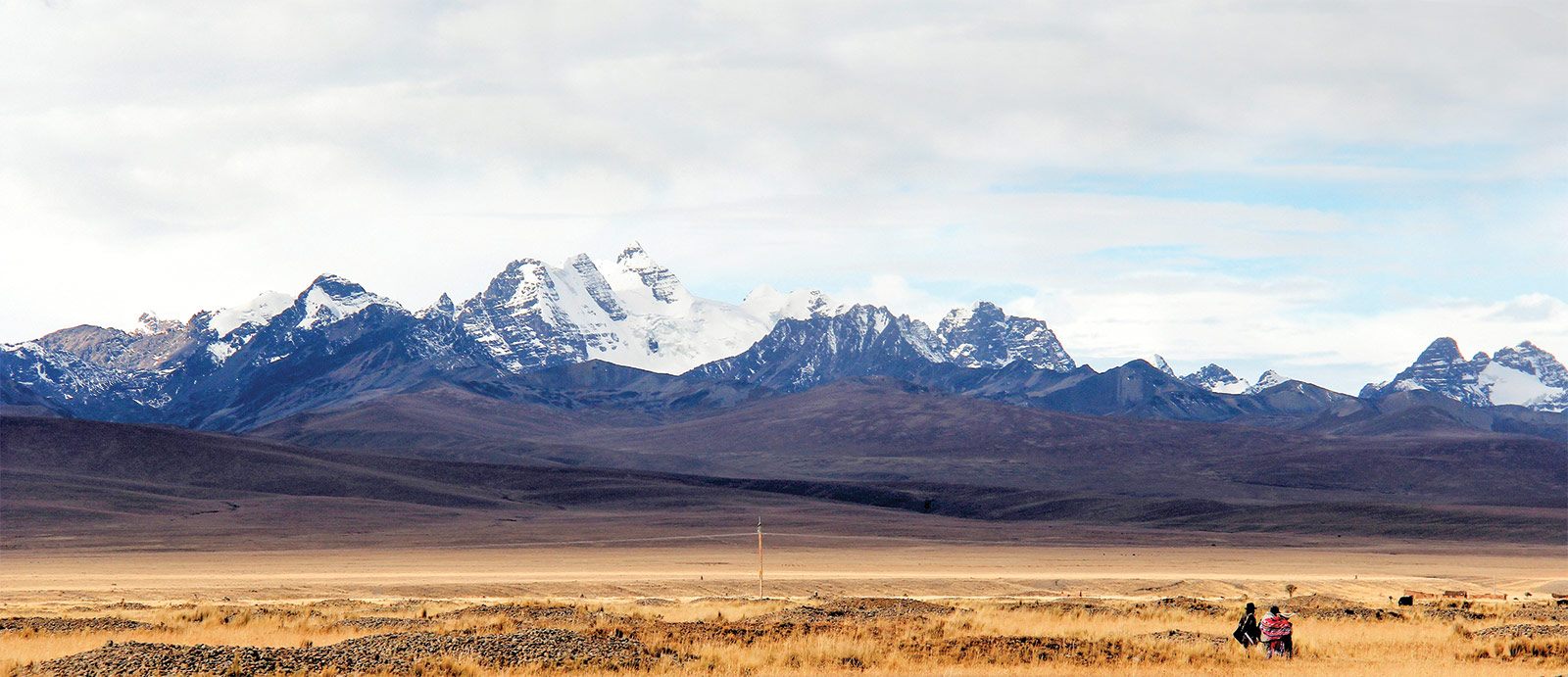
From the seafloor to the tallest mountain range, every landform on Earth has a story to tell that stretches back over geologic time. Earth’s geologic wonders have been shaped over hundreds of millions of years by the movement and interaction of a set of tectonic plates that make up the outer layer of Earth. While scientists can speculate about plate motion, the geodynamics responsible for a wide variety of landforms and events is complex to teach and learn.
Earth science teachers can explain specific phenomena along plate boundaries, but they also have the difficult task of helping students develop a systems-level conceptual understanding of plate tectonics. This big-picture reasoning includes multiple events and processes taking place simultaneously around the globe, and it requires students to recognize what happens at plate boundaries as well as how each tectonic plate is surrounded by—and interacts with—adjacent plates at the same time.
The theory of plate tectonics explains the formation and global distribution of geological phenomena. It is a unifying theory in Earth science. Through the movement and interaction of tectonic plates, material circulates back and forth between
Earth’s crust and the mantle—the layer between the crust and the outer core.
The Geological Models for Explorations of Dynamic Earth (GEODE) project, funded by the National Science Foundation, is developing new geodynamic plate tectonic modeling software for middle school Earth science classrooms that allows students to observe and describe the formation of surface geologic features in terms of plate interactions. The goal of the interactive modeling environment and curriculum is to help students reason spatially and temporally about how plate movements result in the global distribution of various geological phenomena, such as undersea mountain ranges, deep ocean trenches, dramatic volcanic mountains, and vast plains. The software features a system of plates bounded on all sides by other adjacent plates, which interact like those found on Earth.
Earth’s surface features
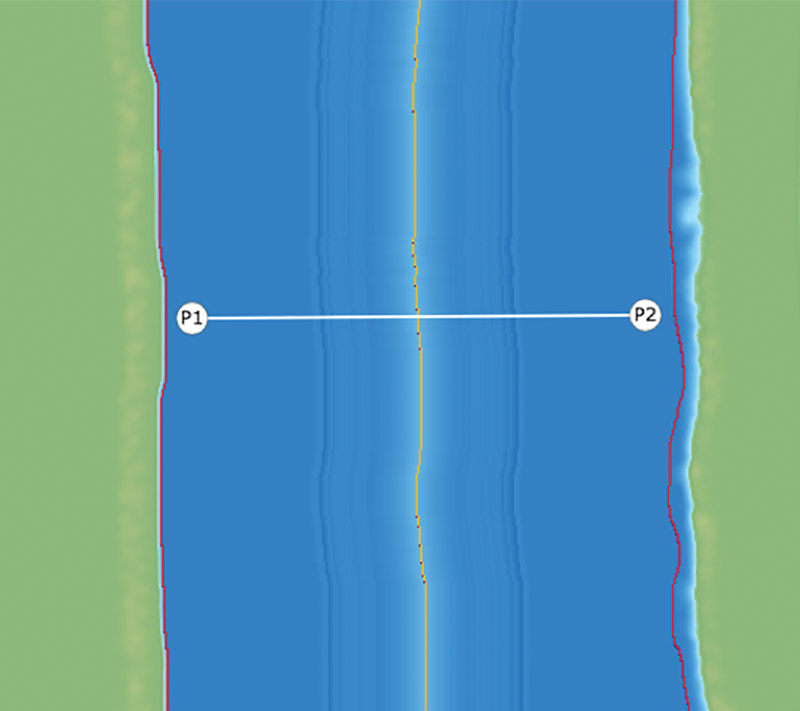
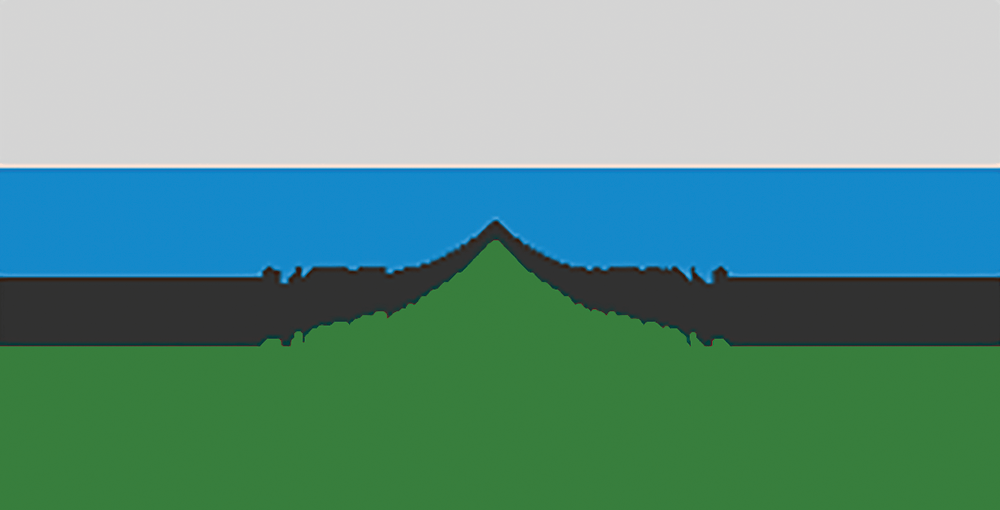
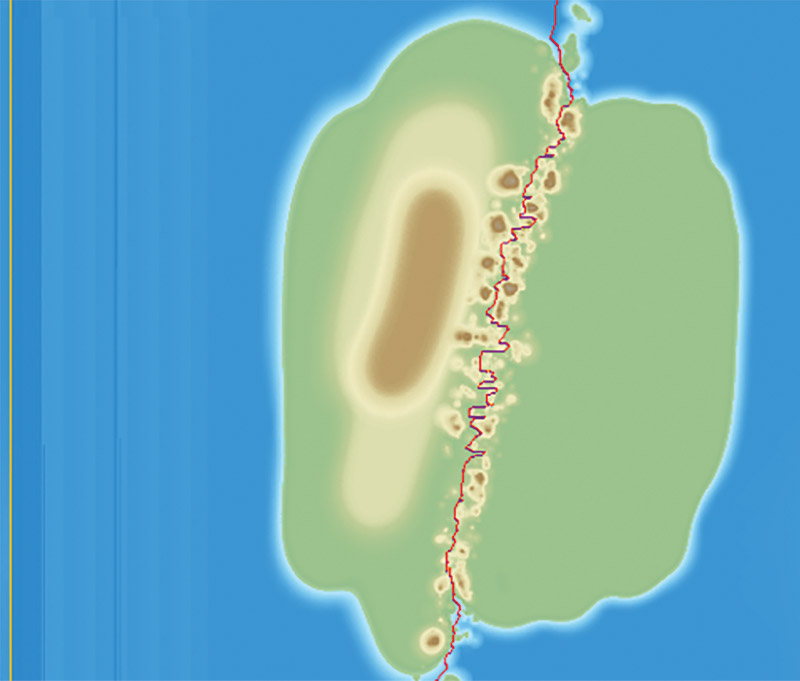
We began software development by modeling divergent boundaries, locations where plates are moving away from each other. Imagine a giant conveyor belt moving two plates apart. Eventually, an underwater volcanic mountain range forms along that divergent boundary from magma that rises from deep within the Earth.
One of our first prototype models is this type of ocean-spreading ridge. A top view (Figure 1) looks down from above at the spreading ridge. Figure 2 shows a cross-section of the divergent boundary of Figure 1. When the model is running, the student sees the movement of the plates, the addition of new crust at the ridge, and the subsequent variation in topography as the underwater mountain range forms. Topographical differences are represented in the top-view model as variations of blue. In the cross-section view they appear as a jagged surface.
The model also shows the crust sinking and getting thicker as it moves away from the spreading ridge, representing the increased density of the cooling crust as it shifts. But even in this simple model we need to consider many design issues. How tall should the mid-ocean ridge be in the cross-section? How do we model the jagged pattern typically found along the mid-ocean ridge caused by faulting. Can we show the magma reaching the surface? How do we make it clear that the layer below the surface is not liquid but solid rock at a temperature just below its melting temperature? (It flows more like road tar than magma.)
Similar issues emerge when modeling convergent boundaries where plates are moving toward each other. Along convergent boundaries, the plate with the denser crust is dragged below the plate with the less dense crust, a process called subduction. At an ocean-continent boundary, the denser oceanic crust subducts beneath the less dense continental crust. At a continent-continent boundary, however, the situation is more complicated.
The model must be able to show how two continents once separated by an ocean collide at a convergent boundary. First, the oceanic crust subducts, bringing the continents closer to each other, with volcanoes forming on the overriding plate. When the continent on one plate reaches the boundary and cannot subduct, it collides with the continent on the other plate, forming mountains (like the Himalayas) along the boundary (Figure 3). But those are just the surface features. The heights of mountains are matched by equivalent “roots” below the mountains. A cross-section view must also show this. Additionally, the model must take into account the collision of two continents, since the plate motion slows down and then stops, which in turn affects the motion of all other plates on the planet.
Multiple boundaries and interactions
Our goal is to develop a geodynamic model showing multiple interacting plates so students can observe interactions that are occurring at different places at the same time. After all, if one side of one plate is diverging from a second plate, then the opposite side of the first plate must be converging on another plate.
Students will be able to create different starting conditions on an imaginary planet with multiple plates interacting in similar ways to tectonic plates on Earth. This will allow them to explore the dynamic interactions along plate boundaries and associated geologic phenomena by changing the planet’s conditions. Students will learn how plate motion has shaped the configuration of Earth’s continents, and be able to explore the evolution of the surface of a planet and how its features change over time. The GEODE curriculum will help them understand where volcanic arcs are located, why there are different patterns of earthquakes around the world, and how supercontinents are formed and separate. Understanding these phenomena will enable students to make reasonable predictions about the future of Earth’s surface features.
By helping students recognize the interconnectedness of Earth’s dynamic plate system, GEODE aims to foster reasoning about changes that occur over geologic time. Earth science is traditionally taught largely in a descriptive manner with videos, text, and non-interactive simulations. Modeling holds huge potential for transforming Earth science education by supporting students’ understanding of complex dynamic systems.
Amy Pallant (apallant@concord.org) directs the GEODE project.
This material is based upon work supported by the National Science Foundation under grant DRL-1621176. Any opinions, findings, and conclusions or recommendations expressed in this material are those of the author(s) and do not necessarily reflect the views of the National Science Foundation.