Engineering Energy Efficiency with a Green Building Model Kit
Precollege engineering education is increasingly recognized as an indispensable part of STEM education. The National Research Council’s conceptual framework for new science education standards has concluded that engineering should be incorporated into American science education.* When the new standards are finally made, thousands of science teachers will be charged with teaching engineering — a subject that may be new to many.
At the center of engineering education is the question of how to teach engineering design. Engineering without design is like science without inquiry. It is through the cycle of designing, testing, and modifying that students engage in authentic engineering practices and learn proven engineering principles. A classroom engineering project without a design challenge for students to make a product is incomplete at best. But the variety of engineering systems precollege students can realistically design, build, and test in classrooms is limited by time, resources, and student preparedness.
Robotics and computer programming are perhaps the most frequently adopted student projects. To cover a wider spectrum of science and allow for broader and deeper infusion of engineering, more options are needed. Situated in the context of sustainability and centered on the concept of energy, our Green Building Model Kit adds to the family of engineering design projects.
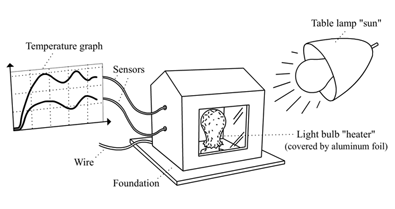
An engineering kit
The Green Building Model Kit was developed to support our Engineering Energy Efficiency curriculum for high school engineering. The curriculum bridges science and engineering by combining scientific inquiry with engineering design. Through laboratory experiments and computer simulations, students are guided to learn the science behind energy flow and energy usage in houses. Having acquired the basic knowledge and skills necessary to undertake more sophisticated tasks, they then team up to design, construct, test, and improve a model house step-by-step using the kit, with the goal of maximizing the house’s energy efficiency. The curriculum has been improved through several rounds of field tests involving more than 300 high school students in Massachusetts. The majority of students surveyed have indicated that creating a model house and measuring its energy performance using the kit was easy.
The Green Building Model Kit uses inexpensive tools and materials, so it can be easily implemented in precollege classrooms. For example, a 40 W light bulb is used to simulate a furnace—it has low heat capacity and can reach a high temperature rapidly, which allows it to warm up a model house in a short time. Wrapped with aluminum foil that has low emissivity, the light energy it radiates heats the foil and the air around it, mimicking the heat transfer through air circulation from a furnace. A 300 W light bulb in a gooseneck fixture simulates the sun at different angles in different seasons. The kit includes fastresponse temperature sensors for testing each design and modification, and students are able to repeatedly assess whether their designs improve energy efficiency.
We have also developed two free software tools that enhance the kit. Energy3D is an educational computer-aided design (CAD) tool that can be easily used to sketch up buildings and print them out for scale-up and assembly using cardstock or foam core. Energy2D is an educational computational fluid dynamics (CFD) tool that provides interactive simulations for studying basic concepts in thermodynamics and heat transfer, such as heat capacity, conduction, convection, and radiation.
Engineering design
The richness of design is the key to successful engineering projects as the vast success of robotics and programming projects has demonstrated. The Green Building Model Kit allows for two design phases. In the first phase, students design and build their own model houses. In the second phase, they design and add energy-efficiency measures to their houses. The kit is flexible enough for different classroom implementations. Students can design their own house, and then add green features to reduce energy usage. Or, to save time, teachers may choose to have students make a model house made from a cardstock template more efficient. Improving the energy efficiency of a standard model house provides the same starting point and constraints for every team.
To make their houses more energy efficient, students might add insulation, sealing, or passive solar units. Each of these features is an application of one or more concepts in power, energy, and heat transfer. For example, insulation reduces energy loss through heat conduction while air sealing prevents infiltration through thermal convection. Both insulation and air sealing achieve energy efficiency by cutting heat loss. Passive solar units, on the other hand, conserve energy by harnessing energy from the sun. Using our kit, students can design different ways to harvest solar energy for supplemental heating.
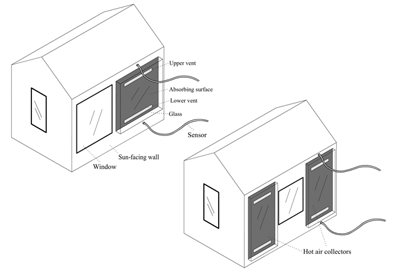
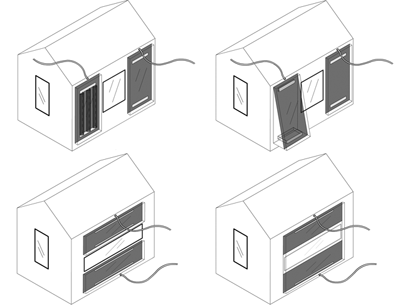
Designing a solar hot air collector
Passive solar architecture studies the interactions between a building and solar radiation, with the goal of finding an optimal way to collect as much solar energy as possible for heating the building in winter. These interactions can be modeled using our kit. The design of a hot air collector (HAC) demonstrates this.
A hot air collector consists of a light-absorbing dark surface, an air space enclosed by glass, and two vents (Figure 2a). Sunlight shines through the glass and heats up the dark surface. A collector transfers the absorbed heat to the house in two ways. First, the heat conducts into the wall behind the surface and is then radiated into the house. Second, the heat warms the air near the surface. The hot air rises and enters the house through the upper vent, which creates an updraft force that draws the cooler air at the bottom of the house into the air space through the lower vent. The air in the house can, therefore, circulate through the HAC and get heated. This circulation effect is sometimes called thermosiphon.
Hot air collector units are usually mounted to the sun-facing wall, which also has windows to let light in. If you think of this design challenge as a task to capture as much energy from the sun as possible, it is really a problem about the optimal use of the sun-facing wall surface. What’s the best way to use this precious house surface area?
This example is fascinating because it involves the synthesis of knowledge about all three mechanisms of heat transfer. Students can easily make a hot air collector and add it to a model house. The variety of designs they can invent and test is limited only by their imagination. Figure 2 shows two possible layouts of hot air collectors and windows. For the design shown in Figure 2a, students can compare the energy gains and losses through a window and a hot air collector. Figure 2b shows an alternative design with two HAC units when, for other design constraints (for example, aesthetics), the window must be located in the middle of the wall.
Figure 3 shows four additional variations. Figure 3a illustrates the idea of corrugating the absorbing surface to enlarge the interface for heat exchange between the air and the surface. Although an interesting idea, the design does not increase solar input. Figure 3b shows a design in which the absorbing surface slants to receive more sunlight, similar to a solar hot water collector. Figure 3c aligns two HACs and a window vertically while Figure 3d improves the energy efficiency by combining the benefits of windows and HACs. With a large HAC unit with the middle part replaced by a window, sunlight can still shine into the house through the window. As the HAC unit is tall, the convective heat flow into the house is more significant.
The hot air collector examples demonstrate the ability of the Green Building Model Kit to support creative engineering design. Students can also design many variations of sunspaces, such as sunrooms, solariums, and greenhouses. Some of these capabilities will be added to our Energy3D design software to enable the exploration and evaluation of various designs before making real products. All these design capacities are critically important to engineering education as they hold the promise of reducing the tendency of “cookbook” design in the classroom. By empowering students to think and design in many different ways, precollege engineering education will blossom.
Charles Xie (qxie@concord.org) directs the Engineering Energy Efficiency project.
Ed Hazzard (ehazzard@concord.org) is a senior science curriculum developer.
Saeid Nourian (snourian@concord.org) is a senior programmer.
This material is based upon work supported by the National Science Foundation under grant DRL-0918449. Any opinions, findings, and conclusions or recommendations expressed in this material are those of the author(s) and do not necessarily reflect the views of the National Science Foundation.