How Can Less Be More?
Phil Morrison first advanced the hypothesis that “less may be more.” In 1963 he was one of an MIT threesome who introduced the idea of science education reform by creating PSSC Physics. He thought students could learn science better by concentrating on a few ideas “to break with the [deductive] Euclidean model … to go beyond mere verbal and formula-learning.”1
Forty-five years later, science curriculum has gone in the opposite direction, and is famously said to be “a mile wide and an inch deep.”2 Standards and tests demand such a comprehensive range of topics that some courses introduce more vocabulary than foreign language courses. In the rush to cover all the required topics, few students learn real science, just the facts and superficial ideas needed by tests.
The technology helps students understand by allowing them to experience inaccessible phenomena and experiment with unrealistic systems.
The antidote is to dig more deeply into fewer topics, and focus on powerful concepts that students discover through guided exploration enabled, as needed, with technology. Students should be able to apply the resulting deeper conceptual understanding to a wide range of topics, making it possible to create a curriculum that is both deep and wide.
An example of going deeper
Most science students must memorize compartmentalized facts about such topics as Kinetic Molecular Theory, latent heat, thermal expansion, and so on. When I was a student, I had to memorize the equation of an ideal gas, PV=nRT. We did experiments with compressing gases that helped me remember the facts and learn to use the gas law equation, but this did nothing to explain where it came from.
Boiling temperature and latent heats were more accumulated facts. I learned that even if you turn up the heat, water boils at a single temperature; this and the melting temperature are so stable that they form the basis of the Celsius scale. These phenomena are somehow related to the fact that it takes energy to convert liquid water into vapor and ice to water. Again, no explanation was given, no connections made to other ideas. These and other facts about the world accumulated in multiple, disconnected areas: thermal expansion, evaporation, diffusion, crystals, conductivity, and more. Science was reduced to learning facts, which results in a completely erroneous image of the nature and conduct of science.
Instead, science should be about unifying concepts and explanations about how the world works. It should not be a catalog of disconnected observations. By going a bit deeper, all of these phenomena can be united through three simple principles:
1. Atoms and molecules find one another repellant—it is very hard to squeeze them together.
2. Atoms and molecules are sticky—they attract when they are close but not touching.
3. Atoms and molecules have no friction. They have no way to dissipate energy, so energy is conserved.
It is not enough to simply state these principles. Students need an opportunity to develop an intuitive understanding of them and build mental models of these kinds of interactions. This cannot be done in the lab. A highly interactive simulation is needed that can allow students to play around in a world of atoms and molecules in order to gain a feel for this peculiar world. This is the very reason we developed the Molecular Workbench.
The Molecular Workbench to the rescue
Molecular Workbench guides students through a series of observations and discoveries that link basic properties to all the phenomena described above: gas laws, phase change, thermal expansion, and more. It allows students to experiment with different kinds of atoms, molecules, and mixtures. Atoms can be made huge or tiny, massive or light, energetic or still. The interaction of a pair can be examined in detail, or the emergent behavior of hundreds observed as a group.
To understand the gas laws, for instance, students need to understand how pressure and temperature manifest at the atomic scale. Molecular Workbench experiments demonstrate that temperature is simply the average kinetic energy of atoms. They show that pressure is the average force exerted by large numbers of atoms hitting a wall. With these insights, the gas law is easy to understand.
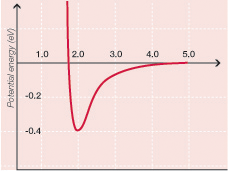
Is digging worth it?
What is the value of knowing that observables like the gas law actually depend on the properties of atoms? Does it help students remember facts such as the gas law? Or does going deeper simply lengthen the list of things students have to memorize?
The answer requires a different perspective. Observables like the gas law are important only because they are related to more fundamental properties of matter. Tests focus on student ability to recall facts about the observable world and to solve numerical problems using simple algebraic equations. But this is not science. The science is in the interconnectedness, the logic of why the world is the way it is.
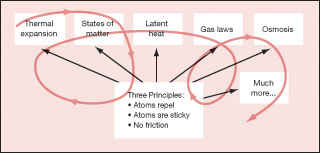
Why stop digging?
For students with a bit more sophistication, the three principles stated above can be introduced with the Lennard-Jones potential shown in Figure 1. This graph shows how the potential energy between two atoms depends on how far apart the atoms are. The Lennard-Jones potential may be a more compact and quantitative way of stating the three principles, but it still does not explain where the forces come from. For students able to dig even deeper, the attraction between atoms can be seen as the result of a kind of polarization of the electron clouds and the repulsion as a consequence of the Pauli exclusion principle. These, in turn, can be derived from basic quantum mechanics concepts.
So when should we start teaching these ideas to introductory students? It would be absurd to start at the deepest level with quantum mechanics, but it is equally absurd to never dig deep. A spiral approach seems more logical, starting with a few easily observed phenomena and then introducing an atomic-scale explanation. The red line in Figure 2 suggests how a spiral might start with observations, link to basic principles, and then spiral back to more observations and additional atomic-scale insights.
Going deeper in other topics
Molecular Workbench can give students access to ideas that are normally thought to be too abstract and inaccessible for introductory students. The technology helps students understand by allowing them to experience inaccessible phenomena and experiment with unrealistic systems. The resulting learning is conceptual, but sufficiently robust to be transferred to new situations.
Using technology to provide experience with otherwise inaccessible concepts can be applied in many science topics. BioLogica simulates the genetics of organisms and can be used for student experimentation on breeding, genetic drift, natural selection, and evolution. Similarly, interactive simulations of gravitating objects, colliding plates, structures that can break, and chemical reactions can all help students understand basic ideas behind observable phenomena. Not all these simulations currently exist, but one can imagine that when they do, science education will be forever changed, giving more students access to the powerful concepts that make science the exciting adventure that it is.