Intermolecular Interactions are Key to Understanding
Have you ever played the game where the goal is to determine how a series of items is connected?
Try this:
- Why does water form on the side of a cold glass?
- Why does global warming mean there will be bigger and more powerful hurricanes?
- How can a tiny change in a gene cause a major disease like sickle cell anemia?
The answers to all of these questions can be found in how molecules attract to each other. This one foundational concept can link what appear to be disparate topics into a coherent framework for understanding the world around us. Indeed, much of what we observe can be explained as emergent phenomena based on the interactions of atoms and molecules.
Richard Feynman, Nobel laureate in physics, said that if he could only pass on a single concept to a world bereft of scientific knowledge it would be the atomic hypothesis: “that all things are made of atoms—little particles that move around in perpetual motion, attracting each other when they are a little distance apart, but repelling upon being squeezed into one another.” From this idea we can begin to explain the answers to the questions above.
The Interactions project, funded by the National Science Foundation as a partnership between the Concord Consortium, Michigan State University’s CREATE for STEM Institute and the University of Michigan, is developing a semester-long interdisciplinary science course based on this fundamental concept. Four units cover intermolecular interactions from various macroscopic and submicroscopic perspectives, providing the foundation for subject-specific courses that follow—from physics to chemistry and biology.
Unit 1: Why do some things stick together while other things don’t?
Students explore basic electrostatic attractions between macroscale objects, then drill down to the atomic level to understand the particulate nature of matter and how atoms provide an underlying mechanism for many phenomena.
Unit 2: How can a tiny spark cause a huge explosion?
Students look at electrostatic attractions and energy transformations involved in chemical reactions. They move from studying single atoms to more complex groupings as molecules form.
Unit 3: Why don’t all liquids boil at the same temperature?
Students investigate the structure of molecules and the electronic distribution within them to understand bulk properties of materials, like boiling point, solubility and viscosity that are due to intermolecular attractions.
Unit 4: How do my genes really work?
Students combine everything they’ve learned about molecules, attractions and energy, and apply this to understanding the biochemical nature of life, including everything from the formation of the first cell to DNA replication and protein function.
The units engage students in the practices of science as outlined in the Next Generation Science Standards, including developing and using models, analyzing and interpreting data and engaging in argument from evidence. Curricular units incorporate research-based methods for engagement and exploration.
Coherence
Research has shown that coherence of curriculum is a dominant predictor of student learning.1 By using the atomic world as a lens for understanding the macroscopic world we experience, science becomes a more connected whole. Instead of memorizing many specific rules tailored for understanding particular phenomena, students build a framework based on fewer fundamental scientific concepts and apply that framework widely.
Contextualization
Each Interactions unit is based on a driving question, providing students with a meaningful context for exploration. A good driving question has the following characteristics:2
- Feasibility: Students should be able to design and perform investigations to answer the question.
- Worth: Questions should deal with rich science content and process that match district curriculum standards.
- Contextualization: Questions should be anchored in the lives of learners and deal with important, real-world questions.
- Meaning: Questions should be interesting and exciting to learners.
- Ethical: Questions should not harm living organisms or the environment.
- Sustainability: Questions should sustain students’ interest for weeks.
The classroom maintains a Driving Question Board with the driving question, as well as additional related questions generated by students. To help answer those questions, students generate data, including results from hands-on experiments, annotated computational models, student illustrations of consensus models developed by the class and everyday observations from inside and outside the classroom, and post these data to the board. The teacher’s role is to foster an environment of collaboration, active discussion and student discovery.
Multiple representations
The curriculum links hands-on experiments to Molecular Workbench models, allowing students to explore the same phenomena at different scales. Because atoms are too small to experience firsthand, the abstract world of molecular interactions and motions can be hard for students to grasp. The Molecular Workbench computational modeling platform provides a concrete visualization of the atomic realm and a way for students to see the results of experiments at this level; students get a feel for how atomic-level behavior results in the emergent properties of the systems they experience at the macroscopic level.
For example, when students mix liquids to explore the particle theory of matter, they do both a hands-on experiment and a virtual one (Figure 1). By marrying the macroscopic world of students’ everyday experience with the submicroscopic world, the curriculum helps bridge the abstract and the concrete, forming links to the underlying atomic interactions that connect so many different phenomena.
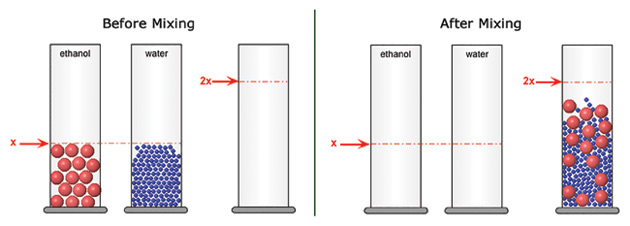
Answer key
So, how do intermolecular attractions provide an explanation for the questions posed at the beginning of this article?
Why does water form on the side of a cold glass?
Air contains many separate water molecules, all attracting each other. If they move fast enough they can overcome those attractions and remain a gas mixed in with the air. When water molecules touch the cold glass, they slow down enough that the attractive forces between them cause them to condense on the side of the glass.
Why does global warming mean there will be bigger and more powerful hurricanes?
Warmer air evaporates more water molecules. During evaporation, heat energy from the air is absorbed by liquid water molecules, which use that energy to overcome their intermolecular attractions. That same energy is released when the water molecules condense back into liquid droplets. The energy released during condensation is what powers hurricanes. More evaporation results in more condensation, resulting in bigger and more powerful hurricanes.
How can a tiny change in a gene cause a major disease like sickle cell anemia?
Genes are a blueprint for making proteins (a type of large, complex molecule). One of those proteins is hemoglobin, which carries oxygen in our blood cells. There is a gene mutation that results in a small change in the hemoglobin protein, causing hemoglobin molecules to stick to each other, forming fibers that distort blood cells into a sickle shape.
Intermolecular attractions are key to understanding these seemingly dissimilar phenomena … and a great deal more.
For too many people science just doesn’t make sense: it seems like a collection of unconnected facts that are difficult to apply to everyday experiences. Our goal is to help students achieve a level of confidence in their ability to learn and apply science, to experience what it means to do science and to begin their journey in using atomic-level reasoning to achieve a deeper understanding of the world in which we live.
1Schmidt, W. H., Wang, H. C., & McKnight, C. C. (2005). Curriculum coherence: An examination of US mathematics and science content standards from an international perspective. Journal of Curriculum Studies, 37(5), 525–559.
2Krajcik, J., & Mamlok-Naaman, R. (2006). Using driving questions to motivate and sustain student interest in learning science. Teaching and learning science: An encyclopedia, 317-327.
Dan Damelin (ddamelin@concord.org) is a technology and curriculum developer.
This material is based upon work supported by the National Science Foundation under grant DRL-1118671. Any opinions, findings, and conclusions or recommendations expressed in this material are those of the author(s) and do not necessarily reflect the views of the National Science Foundation.